The hunter-gatherers learnt to farm. What are the farmers learning?
For more than 90% of human history, we’ve been hunter-gatherers. As nomads with no true home, we roamed the hills and shallows of the prehistoric Earth in small groups, hunting what was needed and gathering as we went.
Then, around 11,000 years ago, we created agriculture.
The reasons why are widely debated, and range from population growth to climate change, but at the end of the day — or rather, the beginning of it — we began to plant seeds and tubers in the ground. We nurtured them until they grew into full plants with juicy fruits and rich tubers, learning along the way that they could produce more when we fed and watered them.
Fertilization. Protection. Irrigation. Over a thousand years, we made leaps and bounds in our knowledge, gaining complete control over nearly every aspect of a plant’s life cycle.
But there was one last one we had yet to get into, and that made perhaps the greatest impact on the quality of food our agriculture produced: breeding.
Plants multiply in many ways. They can clone themselves along horizontal stems like strawberries, or underground stems like potatoes and bamboo. Certain trees, like aspen or cottonwood, can even grow back strong from a lonely branch planted in the ground.
But these methods of multiplication don’t involve pollination, so we won’t get into them right now.
Pollination is how a majority of plants and trees reproduce. From avocados to tomatoes, planting a seed into good, fertile soil is what we all grow up thinking of as gardening — and for good reason. It’s a method of reproduction that we’ve spent millenia perfecting.
It begins with the flower, which, generally, has two parts: the pistil, or female sexual organ,and stamen, or male sexual organ. Stamens hold the pollen, while at the base of the pistil lays the plant’s ovule.
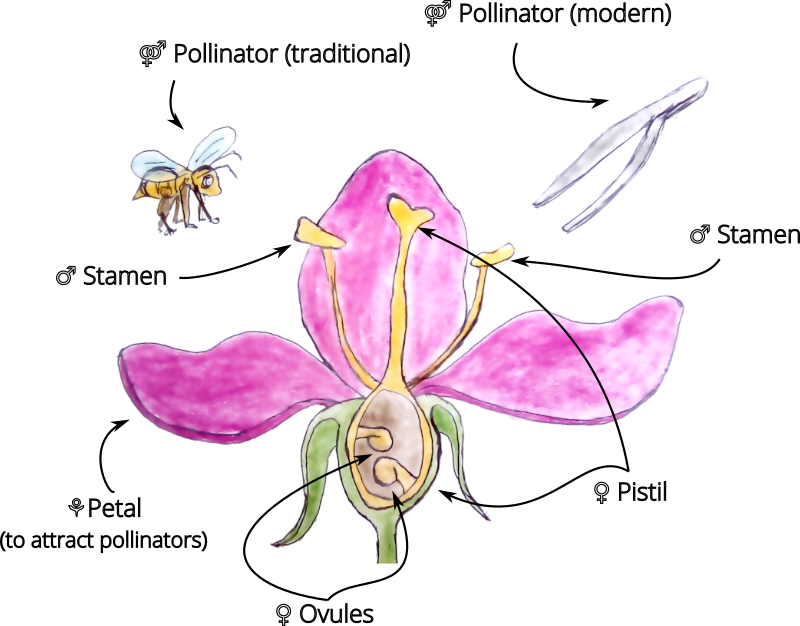
Pollen can be transported across flowers by the wind or by insects to meet a pistil, and eventually fertilize it. Later, the fertilized ovule will grow into a seed, while its surrounding will become a fruit — that’s how it happens in nature.
When a human being jumps into the mix, it isn’t that much different. They just replace the wind.
But it also is different, in that they’re making a choice. The wind doesn’t care which kinds of seed it mixes with what. When humans do pollination by hand, they’re usually trying to create a plant that’s somehow better — taller, stronger, or larger. They’d select two ‘parent’ plants with desirable characteristics, like a high-yielding plant and a disease-resistant plant, and cross them to obtain a plant that’s both highly productive and resistant to a disease.
That’s if they’re lucky. If the new plants inherit the wrong characteristics from each parent, they may well end up being neither productive nor resistant to disease.
This first generation of cross-pollination will yield thousands of plants, but only a few will actually have both the characteristics the breeder wants.
The main genetic theory behind hybrid vigor is called dominance. For each gene existing in a plant, there are many versions of this gene called alleles. Certain alleles are ‘recessive’, or less dominant, and get expressed only if along other recessive versions of the gene. Other alleles are called dominant because they are expressed after masking all recessive alleles. In the dominance genetic theory, each parent brings a set of dominant alleles which complete each other and avoid the possible weaknesses brought by recessive alleles.
This works because each plant actually has two different sets of alleles, or genetic versions: one from each parent. So there are two sets, but only one version of each characteristic can be expressed. A plant can be either tall or short, but not both. (To further complicate things, some plants have more than two sets of alleles — they could have four or eight, which is a big mess for geneticists, but fortunately we don’t have to worry about it now).
Which allele does get expressed is decided by whether it is dominant or recessive. Recessive alleles will only be expressed when both are the same, whereas dominants will trump the other. Continuing with our tall/short example, let’s say that the tall allele is dominant, while the short is recessive. A plant will only grow to be short if the alleles from both parents are short. If even one version is tall, then the plant is automatically going to grow taller.
That means that there’s a one in four chance of getting the exact combination of genes.
Of course, it’s more complicated than this, and there are more factors that affect the process. Hopefully you understand better why it won’t be successful every single time.
So, the handful of plants that are actually successful are then backcrossed with one of the parents for several generations in order to fix its characteristics. At the end of this process, the plant breeder obtains the variety he designed: a high yielding plant resistant to a disease.
Plant breeding is a powerful technique used every day by agronomists and plant breeders to tweak a natural process in the direction they want.
The main problem of this method is its timespan. At each generation of offspring, a diversity of plants are created, not necessarily with the characteristics desired by the plant breeder; that’s why at each generation, the plant breeder must select the few plants with the features she desires. It is only after the 7th generation onwards that all offspring look alike. For instance, breeding a new variety for annual crops like wheat takes 3 to 5 years, and for perennials like fruit trees it takes decades.
While plant breeders were creating new varieties, they quickly noticed that certain hybrids were creating plants with outstanding features, and that such crosses often involved parents from genetically distant groups. Breeding a commercial variety of corn with a far relative from a wild population, for example, generated very vigorous and productive offspring. The growth and yield of the offspring was always better than the parents’ average, or even better than the best parent.
That’s what we mean by hybrid vigor or heterosis.
In the animal kingdom, the mule is a famous hybrid. The mule is a little horse resulting from the mating of a male donkey with a mare, its robustness perfectly suits the hard agricultural work. Unfortunately, animal hybrids, including donkeys, rarely survive. And even if they do, they are often infertile, so they can’t get little mules of their own.
In the plant world, two genetically distinct varieties or plant species can be crossed together. This kind of hybridization is rare but happens even in nature. For example, the Coffea robusta and Coffea arabica came together a century ago and generated a hybrid of Arabica resistant to leaf rust, a widespread coffee disease. Coffee breeders, in turn, used this natural hybrid to develop new varieties of the arabica coffee.
Nowadays, it’s common practice for plant breeders to cross plants from a wild population of a commercial species of plant like wheat, rice, or coffee with their domesticated counterparts. Especially for widely consumed crops, such breeding strategies have increased yield by as much as 50%, contributing a great deal towards helping to feed the growing human population. On the field, it’s easy to see that hybrids are doing better than their parents. They‘re bigger and produce more: they have more vigor.
While plant breeders know what this hybrid vigor looks like on the field, and know more or less how to make such hybrids, they’re still unsure about the mechanisms behind their improved performance. For complex characteristics like yield, which rely on many plant biological processes, there is almost always an apparent vigor. But vigor also doesn’t always happen. A hybrid can have vigor in leaf size in a certain location, but not in another. Features like fruit size may show vigor only when hybrids are stressed, for example under dry or shaded conditions.
Today, plant breeders can observe hybrid vigor on the field, and take advantage of it to produce high yielding plants. But, it is still a bit like magic.
For over 10,000 years, we produced food through agriculture. We planted seeds into the ground for sustenance — and got so good at it that it transformed the course of human history entirely. It was a revolution because we didn’t have to rely on finding food in order to be able to consume it: we created it all ourselves.
And so, with the coming of hybridisation — this increase in humans meddling with the nitty gritty of every step of reproduction — comes another revolution. Now, we no longer have to rely on the kinds of food the plant produces. Rather, we can produce the plant for the kind of food we want.
Pest-resistant potatoes? Done. Drought-compliant coffee? Sure. Bigger, better, juicier strawberries? Absolutely.
With our modern day understanding of testing and genetics, we’ve opened for ourselves a new door in the ways we can influence growth to result in what we want. We don’t have all the answers yet, but we do have the tools to someday look into all of the questions, and the framework through which we can solve them. If we are who we are because of the agricultural revolution, then we’ll be further transformed by the revolution of hybridisation — and who’s to say what happens next?

*We’re hiring! Well, kinda. If you’re someone interested in reading and writing, and want a fun, vibrant team to work with, we’re looking for new editors to take on board. Learn more about it here.*
(Oh, and by the way, we’re looking out for writers too!)